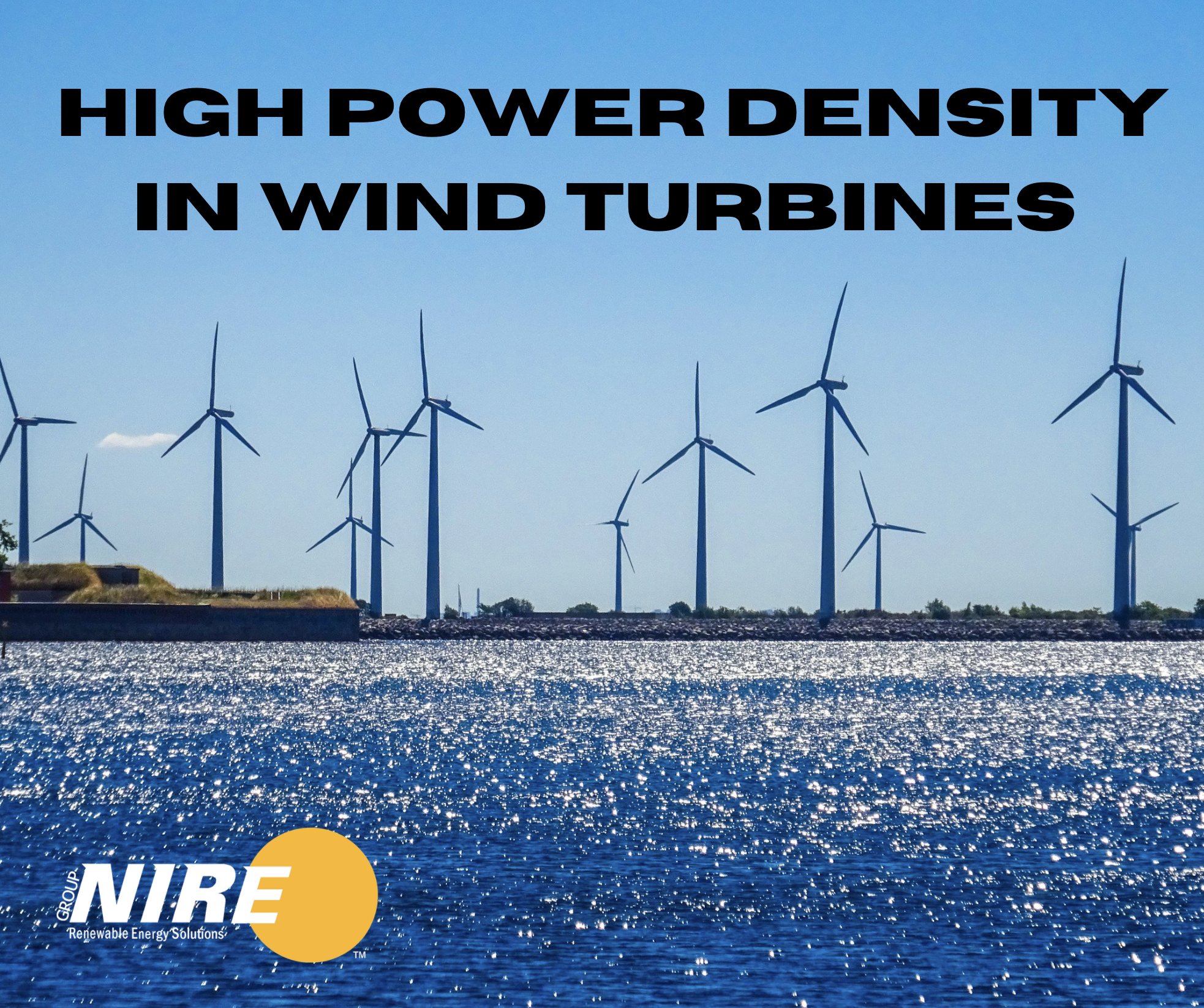
Generators used in wind-turbine systems fall into four architecture types: dc, ac, ac synchronous, and switched reluctance. What are the unique power-density aspects of these versions, including the most widely used among them—the doubly-fed induction generator?

Fundamental physical limits exist as to how much energy can be extracted from renewable resources for a given area of land. There is a wind power density (WPD) definition, not to be confused with power density of system power supplies such as dc-dc converters and dc-ac inverters.
WPD is a quantitative measure of wind energy available at any geographic location. It’s the mean annual power available per square meter of swept area of a wind turbine and is typically calculated for different heights above ground level. To rigorously quantify this, we can calculate an energy source’s power density in watts per square meter (W/m2).
To get an understanding of this idea, designers need to consider the recently opened London Array wind farm to the south of England (Fig. 1). Being one of the world’s largest offshore wind farms, London Array is generating enough energy to power nearly half a million homes. The London Array is displacing about 900,000 tons of CO2 per year. Its total capacity is 630 MW covering a total of 100 km2, and it’s expected to have a capacity factor of 39%. So, the power density of the London Array1 will be 2.5 W/m2.
The United Kingdom is more windy than a good deal of the rest of the world, and some research suggests that large extraction wind farms like this will reduce average power-density numbers closer to 1 W/m2; 2 to 3 W/m2 may be an upper limit on the power density of large-scale wind power. That power density reflects average output. However, peak power density of wind farms can be 3X higher, and minimum power density will be close to zero.
Wind power is one of the most promising renewable energy systems in the 21st century. The largest individual wind-farm capacity in megawatts is located in China. “Wind farms” also can be seen while driving down many highways in the U.S., especially in California, which has second-highest energy capacity deployment in the world, closely followed by India. Germany has the highest wind capacity in Europe at 59.3 GW.
In this article, we will focus on wind-turbine power density in detail, with respect to high-power-density ac-dc generator-side power converters, as well as dc-ac grid-side pulse-width-modulated (PWM) power inverters.
Power Density for Power-Supply Electronics within the Wind Generator
Power electronics enable regulation and conditioning of power, voltage, and frequency with the goal of high efficiency and high power density. Power density is important in the wind turbine especially for efficiency as well as lower weight within the nacelle. The nacelle is about the size of a bus and transforms the slow-turning wind blades into the faster rotor speed.
The generator and gearbox, huge pieces of machinery in the nacelle atop the steel tower, weigh around 56 tons in a 1.5-MW wind generator.
Key Factors that Affect Wind Turbine Generator Output Power
Using power-supply electronics changes the basic characteristic of the wind turbine from being an energy source to an active power source. Electrical technologies used in wind turbines aren’t new, but they have advanced in technology. In today’s market, the price to produce a kWh is so low that solutions with modern power-supply electronics have become very attractive.
Typical wind-turbine generators are induction machines, but they need to produce an ac power output. Thus, they need good dc-to-ac inverter power electronics to convert to a constant frequency that can be connected to the power grid.
Depending on the type of wind-turbine generator (WTG) output, the system may need to use diode-based ac-to-dc rectification converters for an ac generator (see “Types of WTGs” in the next section). Modern power-electronics architectures also use line-commutated thyristor converters as well as self-commutated insulated-gate bipolar transistor (IGBT) and MOSFET converters in wind-power systems.
Self-commutated converters can transfer and control power bidirectionally (both ac-dc and dc-ac) since they can control switches turning off. These inverters integrate electronic switches that are switched on and off directly via the gate, at some number of times per period, using pulse-width-modulated (PWM) control methods; this greatly reduces reactive power consumption and current harmonics. GaN and SiC power devices are also being used as very efficient power switches in this architecture; this will greatly improve power density.
Line-commutated converters are usually used in high power WTG designs; however, they are not able to control the reactive power.
Most commonly used power-electronics converters (PEC) used in wind power are diode and line-commutated converters that have reactive power compensation, diode and PWM voltage source inverters (VSIs), diode and dc-dc chopper and PWM VSI converters, back-to-back PWM VSI converters, and matrix converters.
Types of WTGs
Direct-current (dc) generators
A dc WTG architecture is made up of a wind turbine, a dc generator, IGBT inverter, transformer, controller, and the power grid.
AC synchronous generators
The ac synchronous WTG is a traditional design and accepts dc excitations from permanent magnets known as permanent-magnet synchronous generators (PMSGs)or electromagnets known aselectrically excited synchronous generators (EESGs).
AC asynchronous generators
An ac asynchronous WTG is a modern wind-power system that employs induction machines; these are used extensively in wind-turbine applications. There are two types of architectures:
- Fixed-speed induction generators (FSIGs) with squirrel cage rotors: These generators havethe stator connected to the grid through a transformer, and the rotor is connected to the wind turbine through a gearbox. Until 1998, these generators were usually 1.5 MW or less.
- Doubly-fed induction generator (DFIG) with wound rotors: In modern times, after 1998, more than 85% of installed wind turbines employ the DFIG architecture.
DFIG Architecture
In the popular DFIG system, the power electronic interface controls the rotor currents in order to reach the variable speed needed for maximum energy capture in variable winds. Since the power electronics only process the rotor power, which is typically less than 25% of the overall output power, the DFIG gives the advantages of speed control with reduced cost and lower power losses.
In the DFIG wind turbine, the stator of the generator is directly connected to the grid. The rotor also is connected to the grid, but through a back-to-back (ac-dc to dc-ac) power converter. This speed-adjustable design is typically deployed in power ranges between 1.5 and 6 MW. In a DFIG converter, up to one-third of the power flows through the power semiconductors in both directions.
DFIG Power Circuits

which feeds the generated power into the power grid.
Partial-scale power converter
An electrical power circuit in the DFIG with a partial-scale power converter in a variable-speed controlled wind turbine system is shown in Figure 2. This type of converter performs reactive power compensation with a smooth grid connection. Slip rings and adequate protection schemes are part of this design in case a grid fault occurs.
Full-rated power converter

This power circuit consists of an asynchronous/synchronous generator (Fig. 3). Its architecture eliminates slip rings, has full power capability, and speed controllability as compared with the DFIG architecture. High power loss and switching losses are a part of this architecture.
Switched reluctance generators
These architectures employ a strong rotor and stator. When reluctance generators are combined with direct drive features, these machines would be quite large and heavy, which make them less useful in today’s wind-power applications.
High-Power Wind-Energy-Conversion Systems
In this section, we will discuss back-to-back connected converters. The architecture will be examined for high-power wind-energy-conversion systems (WECS) in the low-voltage category.
The power converter must have high power density to achieve small footprint and weight. This is an important requirement especially for offshore wind turbines.
Low-voltage (LV) converters (690 and 575 V):
Type 4 WECS, back-to-back (BTB)-connected, full-scale, two-level (2L) voltage source converter (VSC) (Fig. 4). The switching frequency of voltage source rectifier (VSR) and VSI is maintained at 1 to 3 kHz to achieve lower switching losses and higher power density.

Type 3 WECS, partial-scale BTB-connected 2L-VSC (Fig. 5). The stator of the generator is directly connected to the grid; the rotor of the generator is connected through the power converter.

Parallel BTB 2L-VSC with common dc-link (Fig. 6). This architecture is for power ratings greater than 0.75 MW in Type 4 turbines (2.5 MW in Type 3 turbines). The current-carrying capability may be increased by connecting the three-phase VSC converters, along with harmonic filters, in parallel.

Parallel BTB 2L-VSCs with individual dc-links (Fig. 7). This architecture solves the issue of circulating currents, as well as the reliability issue; the dc-links can be configured as individual elements. The design preserves the best qualities of power-handling capability, modularity, redundancy, and efficiency.

The fourth listing in References below provides in-depth details and more information regarding the WECS configurations, MW-WECS high-power converters, medium-voltage (MV) converters, passive generator-side converters, converters for multiphase generators, converters for open-winding generators, or power converters without a dc-link.
Read more from thePower Management Series: Delving Into Power Density
References
“Evaluation of Factors affecting Wind Turbine Output Power,” 2017 Nineteenth International Middle East Power Systems Conference (MEPCON), Menoufia University, Egypt, 19-21 December 2017.
“Power converters for wind turbines: Current and future development,” IEEE 2013
“Power Electronics Converters and Wind Turbine,” 2017 International Journal of Engineering Development and Research (www.ijedr.org) (IJEDR), Volume 5, Issue 2, ISSN: 2321-9939
“High-Power Wind Energy Conversion Systems: State-of-the-Art and Emerging Technologies,” Proceedings of the IEEE | Vol. 103, No. 5, May 2015
“Full-Converter Wind Turbine Technology,” Siemens
“Doubly fed induction generator using back-to-back PWM converters and its application to variable-speed wind-energy generation,” IEEE Proceedings-Electrical Power Appl., Vol. 143, No 3, May 1996